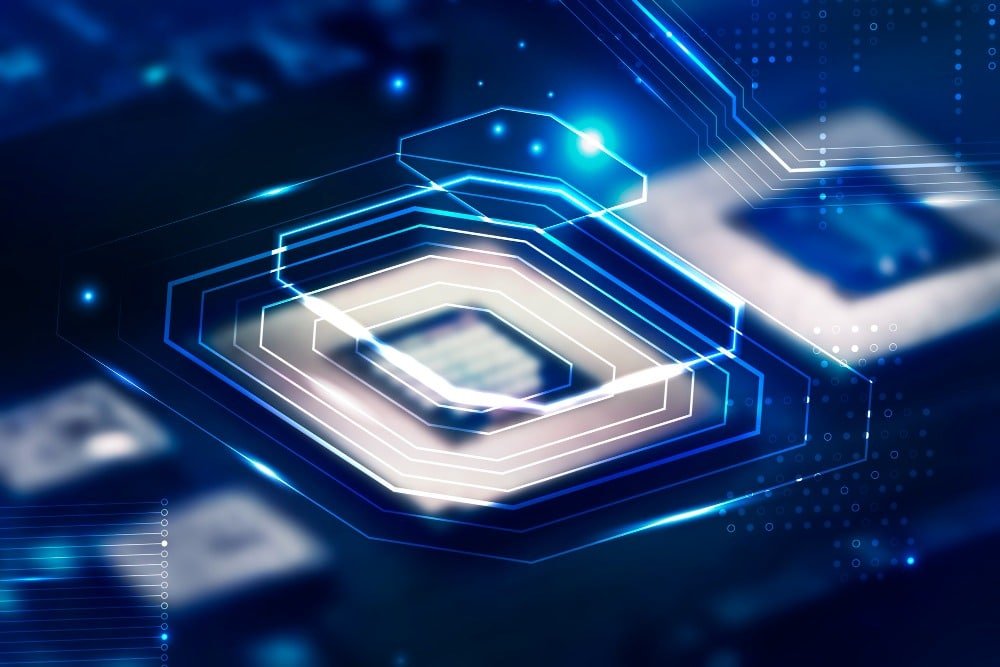
Quantum computing represents a paradigm shift in the realm of computation, harnessing the principles of quantum mechanics to process information in fundamentally different ways compared to classical computers. At its core, quantum computing leverages the unique properties of quantum bits, or qubits, which can exist in multiple states simultaneously, a phenomenon known as superposition. This capability allows quantum computers to perform complex calculations at speeds unattainable by traditional binary systems.
The potential of quantum computing extends beyond mere speed; it promises to revolutionise fields such as cryptography, optimisation, and material science by solving problems that are currently intractable for classical machines. The development of quantum computing is still in its nascent stages, yet it has garnered significant attention from both academia and industry. Major technology companies, including Google, IBM, and Microsoft, are investing heavily in research and development to unlock the full potential of quantum technologies.
As we delve deeper into the intricacies of quantum computing, it becomes evident that understanding its foundational principles is crucial for grasping its implications and future trajectory.
Summary
- Quantum computing harnesses the principles of quantum mechanics to process information in a fundamentally different way than classical computing.
- Quantum mechanics is the branch of physics that describes the behaviour of particles at the atomic and subatomic levels, and it forms the foundation of quantum computing.
- Quantum computing has the potential to solve certain problems much faster than classical computers, particularly in the fields of cryptography, drug discovery, and materials science.
- Quantum computing faces challenges such as maintaining the delicate quantum state of qubits, error correction, and scaling up to practical, large-scale applications.
- The future of quantum computing holds promise for revolutionising industries and scientific research, but it also requires significant advancements in hardware, algorithms, and overcoming limitations.
The Basics of Quantum Mechanics
To comprehend quantum computing, one must first understand the fundamental principles of quantum mechanics. At the heart of quantum mechanics lies the concept of wave-particle duality, which posits that particles such as electrons exhibit both wave-like and particle-like properties. This duality is essential for understanding phenomena like interference and entanglement, which are pivotal in quantum computing.
For instance, when two particles become entangled, the state of one particle instantaneously influences the state of the other, regardless of the distance separating them. This non-locality challenges classical intuitions about causality and locality. Another cornerstone of quantum mechanics is the principle of superposition.
Unlike classical bits that can be either 0 or 1, qubits can exist in a state that is a combination of both 0 and 1 simultaneously. This property enables quantum computers to explore multiple solutions to a problem concurrently, vastly increasing their computational power. The mathematical framework that describes these phenomena is rooted in linear algebra and complex numbers, making quantum mechanics both fascinating and complex.
Understanding these principles is essential for grasping how quantum computers operate and why they hold such promise for future technological advancements.
The distinction between quantum computing and classical computing is profound and multifaceted. Classical computers operate on bits, which are binary units of information that can represent either a 0 or a 1. These bits are manipulated through logical operations to perform calculations and process data.
In contrast, quantum computers utilise qubits, which can represent both 0 and 1 simultaneously due to superposition. This fundamental difference allows quantum computers to perform many calculations at once, leading to exponential speed-ups for certain types of problems. Moreover, classical computing relies on deterministic algorithms that follow a specific sequence of operations to arrive at a solution.
Quantum computing, however, employs probabilistic algorithms that can explore multiple pathways simultaneously. This means that while a classical computer might take an impractically long time to solve a complex problem, a quantum computer could potentially arrive at the solution much more quickly by evaluating numerous possibilities at once. For example, Shor’s algorithm for factoring large integers demonstrates how quantum computers can outperform classical counterparts in specific tasks, such as breaking widely used cryptographic codes.
Quantum Computing Applications
The applications of quantum computing are vast and varied, spanning numerous fields and industries. One of the most promising areas is cryptography. Quantum computers have the potential to break traditional encryption methods that rely on the difficulty of factoring large numbers or solving discrete logarithm problems.
Shor’s algorithm exemplifies this capability, posing a significant threat to current cryptographic systems like RS. However, this also paves the way for new forms of secure communication through quantum key distribution (QKD), which utilises the principles of quantum mechanics to create unbreakable encryption keys. In addition to cryptography, quantum computing holds promise in optimisation problems prevalent in logistics and supply chain management. For instance, companies like Volkswagen have explored using quantum algorithms to optimise traffic flow in urban environments, potentially reducing congestion and improving efficiency.
Furthermore, in the realm of drug discovery and material science, quantum computers can simulate molecular interactions at an unprecedented level of detail. This capability could lead to breakthroughs in developing new materials or pharmaceuticals by accurately predicting how different compounds will interact at the quantum level.
Challenges and Limitations of Quantum Computing
Despite its immense potential, quantum computing faces several significant challenges and limitations that must be addressed before it can achieve widespread practical application. One of the primary hurdles is decoherence, which refers to the loss of quantum coherence due to interactions with the environment. Qubits are highly sensitive to external disturbances, and maintaining their state long enough to perform calculations is a formidable challenge.
Researchers are actively exploring error correction techniques and more robust qubit designs to mitigate these issues. Another limitation lies in the current state of quantum hardware. While there have been remarkable advancements in building qubits using various technologies such as superconducting circuits and trapped ions, scaling these systems to create large-scale quantum computers remains a daunting task.
The complexity involved in interconnecting numerous qubits while maintaining their coherence poses significant engineering challenges. Additionally, developing efficient algorithms that can fully exploit the capabilities of quantum hardware is an ongoing area of research.
Quantum Computing Hardware: Qubits and Quantum Gates
The hardware underpinning quantum computing is fundamentally different from that of classical systems. At the core are qubits, which can be realised through various physical implementations such as superconducting circuits, trapped ions, or topological qubits. Each approach has its advantages and challenges; for instance, superconducting qubits are relatively easy to fabricate but suffer from short coherence times, while trapped ions offer longer coherence but are more complex to manipulate.
Quantum gates are the building blocks of quantum circuits, analogous to classical logic gates in traditional computing. These gates manipulate qubits through operations that change their states or entangle them with other qubits. Commonly used gates include the Hadamard gate, which creates superposition, and the CNOT gate, which generates entanglement between qubits.
The ability to construct complex circuits from these gates enables quantum computers to perform intricate calculations. However, designing efficient quantum circuits that minimise errors while maximising computational power remains an active area of research.
Quantum Computing Algorithms
The development of algorithms specifically designed for quantum computers is crucial for unlocking their potential. Notable examples include Shor’s algorithm for integer factorisation and Grover’s algorithm for unstructured search problems. Shor’s algorithm demonstrates how a quantum computer can factor large numbers exponentially faster than the best-known classical algorithms, posing significant implications for cryptography.
Grover’s algorithm offers a quadratic speedup for searching unsorted databases, showcasing how quantum techniques can enhance data retrieval processes. Beyond these well-known algorithms, researchers are continually exploring new approaches tailored to specific applications. Quantum machine learning is an emerging field that seeks to leverage quantum computing for tasks such as classification and clustering.
By exploiting the unique properties of qubits, these algorithms aim to process large datasets more efficiently than their classical counterparts. As research progresses, it is likely that more innovative algorithms will emerge, further expanding the range of problems that quantum computers can address.
The Future of Quantum Computing
The future of quantum computing holds immense promise but also uncertainty as researchers navigate the complexities inherent in this field. As advancements continue in hardware development and algorithm design, we may witness breakthroughs that bring practical quantum computing closer to reality. The establishment of quantum networks could enable secure communication channels based on QKD principles, fundamentally altering how information is transmitted across the globe.
Moreover, as industries begin to recognise the potential benefits of quantum computing, investment in research and development is likely to increase significantly. Collaborative efforts between academia and industry will be essential in driving innovation and addressing challenges related to scalability and error correction. While it may take years or even decades before we see widespread adoption of quantum technologies, the groundwork being laid today will undoubtedly shape the future landscape of computation and technology as a whole.
In conclusion, while we stand on the precipice of a new era defined by quantum computing, it is essential to approach this field with both excitement and caution. The journey towards realising its full potential will require continued exploration and collaboration across disciplines as we seek to unlock the mysteries of the quantum realm and harness its power for transformative applications across society.
If you are interested in learning more about the legal implications of personal injury fraud, you should check out the article Personal Injury Fraud and Fiction: Desperate Times Call for Desperate Measures. This article delves into the desperate measures some individuals take to commit personal injury fraud and the consequences they face. It provides a fascinating insight into the world of insurance fraud and the legal system’s response to such deceitful practices.
FAQs
What is quantum computing?
Quantum computing is a type of computing that takes advantage of the strange ability of subatomic particles to exist in more than one state at any time. This allows quantum computers to process and store information in a way that is fundamentally different from classical computers.
How does quantum computing differ from classical computing?
Classical computers use bits to process and store information, with each bit representing either a 0 or a 1. Quantum computers, on the other hand, use quantum bits or qubits, which can represent both 0 and 1 simultaneously due to the principles of quantum mechanics.
What are the potential applications of quantum computing?
Quantum computing has the potential to revolutionize fields such as cryptography, drug discovery, materials science, and artificial intelligence. It could also significantly speed up complex calculations that are currently infeasible for classical computers.
What are the challenges in developing quantum computers?
One of the main challenges in developing quantum computers is maintaining the delicate quantum state of qubits, which is easily disrupted by external factors. Additionally, scaling up quantum computers to a practical size and ensuring their reliability and error correction are ongoing challenges.
Is quantum computing accessible to beginners?
While the underlying principles of quantum computing can be complex, there are resources and introductory materials available that can help beginners understand the basics of quantum computing. It is a rapidly evolving field, and there are opportunities for beginners to learn and contribute to its development.