Quantum simulations represent a revolutionary approach to understanding complex quantum systems that are otherwise intractable using classical computational methods. By leveraging the principles of quantum mechanics, these simulations allow researchers to model and predict the behaviour of particles at the quantum level, providing insights into phenomena that are fundamental to various fields, including physics, chemistry, and materials science. The ability to simulate quantum systems opens up new avenues for exploration, enabling scientists to investigate interactions and properties that were previously beyond reach.
The significance of quantum simulations lies not only in their potential to solve existing problems but also in their capacity to inspire new theories and experimental designs. As researchers grapple with the intricacies of quantum mechanics, the development of quantum simulators—devices specifically designed to replicate the behaviour of quantum systems—has emerged as a critical tool. These simulators can mimic the dynamics of complex systems, offering a platform for testing hypotheses and validating theoretical models.
The intersection of quantum mechanics and computational science thus heralds a new era of discovery, where the boundaries of knowledge are continually expanded.
Summary
- Quantum simulations use quantum systems to model and understand complex phenomena in physics, chemistry, and materials science.
- Theoretical foundations are based on the principles of quantum mechanics and the use of quantum computers and simulators.
- Quantum computing and quantum simulators play a crucial role in by manipulating quantum states and simulating quantum systems.
- Quantum simulations have diverse applications in scientific research, including the study of quantum materials, chemical reactions, and complex physical systems.
- Challenges and limitations of quantum simulations include the need for error correction, the scalability and the complexity of simulating large quantum systems.
Theoretical Foundations of Quantum Simulations
At the heart of quantum simulations lies the framework of quantum mechanics, which describes the behaviour of matter and energy at the smallest scales. Central to this framework is the concept of superposition, where particles can exist in multiple states simultaneously, and entanglement, which allows particles to be interconnected in ways that classical physics cannot explain. These principles form the basis for simulating quantum systems, as they enable the representation of complex interactions that govern particle behaviour.
The mathematical underpinnings of quantum simulations are rooted in linear algebra and complex probability theory. Quantum states are represented by vectors in a Hilbert space, while observables correspond to operators acting on these vectors. The evolution of quantum states is governed by the Schrödinger equation, which describes how a system changes over time.
In practice, simulating a quantum system involves solving this equation for many-body systems, which can quickly become computationally prohibitive due to the exponential growth of the state space with the number of particles involved. This challenge necessitates innovative approaches and algorithms that can efficiently approximate solutions.
Quantum Computing and Quantum Simulators
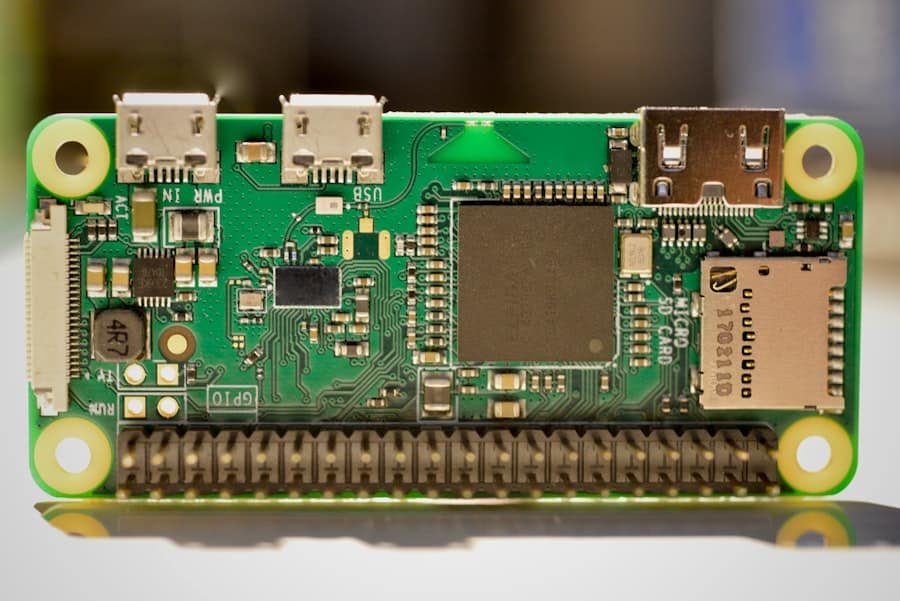
Quantum computing is often conflated with quantum simulations, yet they serve distinct purposes within the realm of quantum mechanics. Quantum computers are general-purpose devices capable of performing a wide range of calculations by manipulating qubits—quantum bits that can represent both 0 and 1 simultaneously due to superposition. This capability allows quantum computers to tackle problems that are infeasible for classical computers, such as factoring large numbers or optimising complex systems.
In contrast, quantum simulators are tailored specifically for modelling particular quantum systems rather than performing arbitrary computations. They are designed to replicate the dynamics of specific Hamiltonians—mathematical descriptions of energy in a system—allowing researchers to explore phenomena such as phase transitions or quantum entanglement in a controlled environment. While both quantum computers and simulators harness the principles of quantum mechanics, simulators provide a more focused approach to understanding specific physical systems, making them invaluable tools in scientific research.
Applications of Quantum Simulations in Scientific Research
The applications of quantum simulations span a diverse array of scientific disciplines, significantly enhancing our understanding of complex systems. In condensed matter physics, for instance, researchers utilise quantum simulations to investigate phenomena such as superconductivity and magnetism. By simulating interactions between electrons in a lattice structure, scientists can gain insights into the conditions necessary for superconductivity to emerge, potentially leading to the development of new materials with enhanced properties.
In chemistry, quantum simulations have transformed our ability to model molecular interactions and reactions. Traditional computational methods often struggle with accurately predicting reaction pathways or molecular geometries due to the complexity of electron correlations. Quantum simulations, however, can provide precise calculations of molecular energies and properties, enabling chemists to design new drugs or catalysts with tailored functionalities.
For example, researchers have employed quantum simulations to explore the electronic structure of complex organic molecules, paving the way for advancements in materials design and drug discovery.
Challenges and Limitations of Quantum Simulations
Despite their promise, they face several challenges that limit their widespread application. One significant hurdle is the issue of noise and decoherence in quantum systems. Quantum states are inherently fragile and can be easily disturbed by their environment, leading to errors in simulation results.
This sensitivity necessitates robust error-correction techniques and fault-tolerant architectures to ensure reliable outcomes from quantum simulations. Another limitation arises from the scalability of current quantum simulators. While small-scale systems can be effectively simulated, as the number of particles increases, the computational resources required grow exponentially.
This phenomenon is often referred to as the “curse of dimensionality,” which poses a significant barrier to simulating larger and more complex systems. Researchers are actively exploring hybrid approaches that combine classical and quantum computing techniques to mitigate these challenges and enhance the capabilities of quantum simulations.
Quantum Simulation Algorithms and Techniques
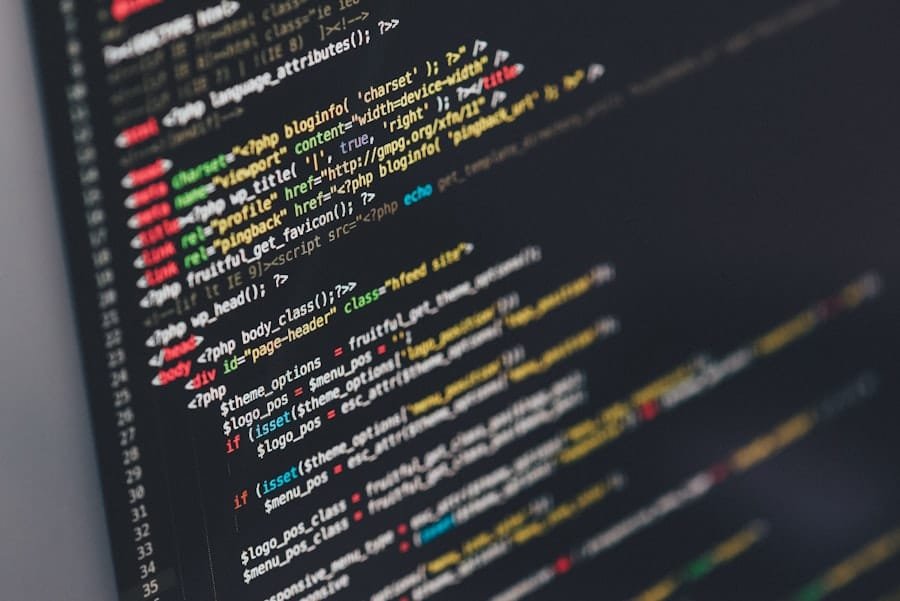
The development of efficient algorithms is crucial for maximising the potential of quantum simulations. One prominent algorithm is the Variational Quantum Eigensolver (VQE), which is designed to find the ground state energy of a quantum system by optimising parameters within a trial wave function. VQE leverages classical optimisation techniques alongside quantum computations, making it particularly suitable for near-term quantum devices that may not yet possess full fault tolerance.
Another notable technique is Quantum Monte Carlo (QMC), which employs stochastic methods to sample from probability distributions associated with quantum states. QMC algorithms can provide accurate estimates of observables in many-body systems by averaging over numerous samples, thus circumventing some limitations associated with deterministic approaches. These algorithms exemplify how innovative computational strategies can enhance the efficiency and accuracy of quantum simulations, paving the way for more extensive applications across various scientific domains.
Quantum Simulations in Materials Science and Chemistry
In materials science, quantum simulations have become indispensable for understanding and designing new materials with tailored properties. For instance, researchers have utilised these simulations to investigate topological insulators—materials that conduct electricity on their surface while remaining insulating in their bulk. By simulating the electronic structure and topological characteristics of these materials, scientists can identify potential candidates for applications in spintronics or quantum computing.
In chemistry, quantum simulations have revolutionised our approach to studying reaction mechanisms and molecular dynamics. The ability to accurately model electron correlations allows chemists to predict reaction rates and pathways with unprecedented precision. For example, researchers have successfully employed quantum simulations to elucidate the mechanisms behind enzyme catalysis, providing insights into how biological catalysts accelerate chemical reactions.
Such knowledge not only enhances our understanding of fundamental biochemical processes but also informs the design of synthetic catalysts for industrial applications.
Future Prospects and Implications of Quantum Simulations
The future prospects for quantum simulations are vast and promising, with implications that extend far beyond current applications. As advancements in quantum hardware continue to progress, we can anticipate an increase in the scale and complexity of systems that can be simulated accurately. This evolution will likely lead to breakthroughs in various fields, including drug discovery, renewable energy technologies, and advanced materials design.
Moreover, as interdisciplinary collaboration between physicists, chemists, computer scientists, and engineers becomes more prevalent, we may witness innovative approaches that integrate quantum simulations with other emerging technologies such as artificial intelligence and machine learning. These synergies could enhance our ability to analyse vast datasets generated by experiments or simulations, ultimately accelerating scientific discovery. In conclusion, while challenges remain in realising the full potential, ongoing research and development efforts are poised to unlock new frontiers in our understanding of complex systems across multiple disciplines.
The implications for science and technology are profound, promising a future where quantum simulations play a central role in shaping our understanding of the universe at its most fundamental level.
The Fundamentals of Quantum Simulations: A New Era of Scientific Discovery explores the groundbreaking advancements in quantum computing that are revolutionizing the field of scientific research. This article delves into the intricacies and their potential to unlock new realms of knowledge and innovation. For entrepreneurs looking to embark on a new business venture, Starting a New Business with Bad Credit provides valuable insights and strategies for overcoming financial obstacles. Setting clear objectives is crucial for the success of any business, as highlighted in the article on Objectives. Additionally, proper wet-dry dog food storage practices are essential for maintaining the quality and freshness of pet food, as discussed in the informative piece on Best Practices for Proper Wet-Dry Dog Food Storage.
FAQs
What is quantum simulation?
Quantum simulation is the use of quantum systems to simulate and understand the behaviour of complex quantum systems that are difficult to study using classical computers.
How does quantum simulation differ from classical simulation?
Quantum simulation differs from classical simulation in that it uses quantum systems, such as quantum computers, to model and understand the behaviour of other quantum systems. Classical simulation, on the other hand, uses classical computers to model classical systems.
What are the potential applications of quantum simulations?
Quantum simulations have the potential to revolutionise fields such as materials science, chemistry, and drug discovery by allowing scientists to study and understand complex quantum systems in ways that were previously impossible.
What are some of the challenges in quantum simulations?
Challenges in quantum simulations include the need for more powerful and reliable quantum hardware, as well as the development of better algorithms and techniques for simulating and understanding quantum systems.
What are some examples of successful quantum simulations?
Successful quantum simulations include the simulation of chemical reactions, the study of exotic materials, and the exploration of quantum phase transitions. These simulations have provided valuable insights into the behaviour of complex quantum systems.