At the heart of quantum computing lies the qubit, a fundamental unit of quantum information. Unlike classical bits, which can exist in one of two states—0 or 1—qubits can exist in a state of superposition, allowing them to represent both 0 and 1 simultaneously. This unique property arises from the principles of quantum mechanics, which govern the behaviour of particles at the atomic and subatomic levels.
A qubit can be realised through various physical systems, including photons, electrons, and even atoms, each exhibiting distinct characteristics that influence their performance in quantum computations. The representation of a qubit is often visualised using the Bloch sphere, a geometrical representation where any point on the surface corresponds to a possible state of the qubit. The poles of the sphere represent the classical states of 0 and 1, while points along the equator represent superposition states.
This visualisation not only aids in understanding the complex nature of qubits but also highlights their potential for parallel processing. By leveraging superposition, quantum computers can perform multiple calculations at once, vastly increasing their computational power compared to classical systems.
Summary
- Qubits are the basic units of quantum information in quantum computing, representing a quantum bit that can exist in multiple states simultaneously.
- Superposition and entanglement are fundamental principles of qubits, allowing them to perform complex calculations and solve problems at a much faster rate than classical bits.
- Qubits offer advantages over classical bits in quantum computing, such as the ability to process and store a vast amount of information and perform parallel computations.
- Building and manipulating qubits require advanced technologies and methods, including superconducting circuits, trapped ions, and topological qubits.
- Quantum algorithms and applications of qubits have the potential to revolutionize fields such as cryptography, drug discovery, and optimization problems.
The Principles of Superposition and Entanglement in Qubits
Superposition is one of the cornerstones of quantum mechanics and plays a pivotal role in the functionality of qubits. When a qubit is in a state of superposition, it can be thought of as being in a blend of both 0 and 1 until it is measured. This measurement collapses the superposition into one of the definite states, either 0 or 1.
The ability to exist in multiple states simultaneously allows quantum computers to explore many solutions to a problem concurrently, making them particularly powerful for certain types of calculations, such as factoring large numbers or simulating quantum systems. Entanglement is another fundamental principle that enhances the capabilities of qubits. When two or more qubits become entangled, the state of one qubit becomes dependent on the state of another, regardless of the distance separating them.
This phenomenon was famously described by Einstein as “spooky action at a distance.” Entangled qubits can be manipulated in such a way that measuring one qubit instantly provides information about its entangled partner. This property is crucial for quantum communication and cryptography, as it enables secure transmission of information that cannot be easily intercepted or tampered with.
The Advantages of Qubits over Classical Bits in Quantum Computing
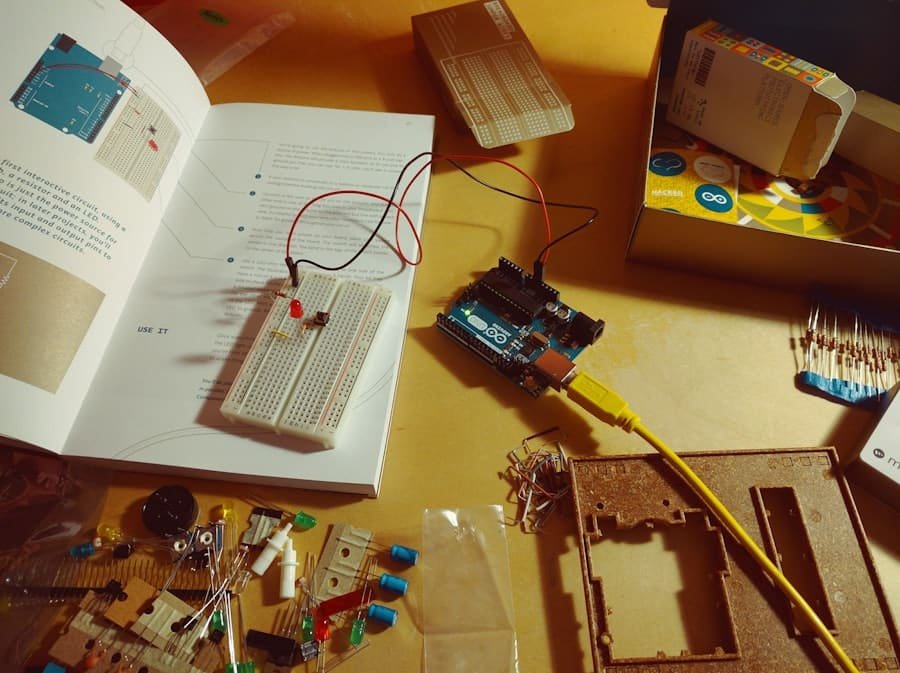
The advantages of qubits over classical bits are profound and transformative for computational capabilities. One significant advantage is the exponential scaling of computational power that arises from superposition. In a classical system, adding more bits increases processing power linearly; however, with qubits, each additional qubit doubles the number of possible states that can be represented.
For instance, while a classical computer with n bits can represent 2^n states, a quantum computer with n qubits can represent all those states simultaneously due to superposition. This exponential growth allows quantum computers to tackle problems that are currently infeasible for classical computers. Moreover, the entanglement property of qubits facilitates complex operations that would be prohibitively time-consuming on classical systems.
Quantum algorithms can exploit entangled qubits to perform tasks such as searching unsorted databases or solving optimisation problems more efficiently than their classical counterparts. For example, Grover’s algorithm demonstrates how a quantum computer can search through an unsorted database in O(√N) time, compared to O(N) time for classical algorithms. This efficiency opens up new avenues for research and application across various fields, including cryptography, material science, and artificial intelligence.
Building and Manipulating Qubits: Technologies and Methods
The construction and manipulation of qubits involve various technologies that harness different physical phenomena. Superconducting circuits are one prominent method used to create qubits. These circuits operate at extremely low temperatures and utilise Josephson junctions to create non-linear inductance, allowing for the creation of coherent quantum states.
Superconducting qubits have gained significant attention due to their relatively high coherence times and scalability, making them suitable for building larger quantum processors. Another approach involves trapped ions, where individual ions are confined using electromagnetic fields and manipulated with laser beams. Each ion acts as a qubit, and their interactions can be controlled with high precision.
Trapped ion systems have demonstrated remarkable fidelity in operations and are capable of implementing complex quantum gates necessary for quantum algorithms. Additionally, photonic qubits utilise properties of light to encode information, allowing for high-speed transmission and potential applications in quantum communication networks.
Quantum Algorithms and Applications of Qubits
Quantum algorithms leverage the unique properties of qubits to solve problems more efficiently than classical algorithms. Shor’s algorithm is one of the most famous examples; it can factor large integers exponentially faster than the best-known classical algorithms. This capability poses significant implications for cryptography, particularly for public-key encryption systems that rely on the difficulty of factoring large numbers for security.
Beyond cryptography, quantum algorithms have applications in optimisation problems prevalent in logistics and finance. Quantum Approximate Optimisation Algorithm (QAOA) is designed to find approximate solutions to combinatorial optimisation problems by exploiting quantum superposition and entanglement. Furthermore, quantum simulations enable researchers to model complex quantum systems that are otherwise impossible to simulate classically, providing insights into materials science and drug discovery.
The potential applications extend into machine learning as well. Quantum machine learning algorithms aim to enhance data processing capabilities by leveraging quantum parallelism. For instance, quantum support vector machines could potentially classify data faster than their classical counterparts by utilising quantum states to represent data points in high-dimensional spaces.
Challenges and Limitations of Qubits in Quantum Computing
Decoherence: A Major Hurdle
One major issue is decoherence, which occurs when qubits lose their quantum state due to interactions with their environment. This loss of coherence limits the time available for performing computations before errors occur.
Scalability: A Formidable Task
Maintaining coherence is crucial for executing complex algorithms; thus, researchers are actively exploring error correction techniques and fault-tolerant architectures to mitigate these effects. Another challenge lies in scalability. While small-scale quantum processors have been developed successfully, scaling up to larger systems while maintaining low error rates remains a formidable task.
Complexity and Standardisation
The complexity increases as more qubits are added; managing interactions between them without introducing noise or errors becomes increasingly difficult. Additionally, the physical implementation of qubits varies widely across different technologies, leading to challenges in standardisation and interoperability among different quantum systems.
The Future of Qubits and Quantum Computing
The future of qubits and quantum computing holds immense potential as advancements continue to unfold across various domains. Researchers are actively working on developing more robust qubit designs that enhance coherence times and reduce error rates. Innovations such as topological qubits aim to create more stable qubit states by exploiting anyonic excitations in two-dimensional materials, potentially leading to fault-tolerant quantum computing architectures.
Moreover, hybrid approaches that combine classical and quantum computing are gaining traction. These systems leverage the strengths of both paradigms to tackle complex problems more effectively. For instance, using classical computers for pre-processing data before feeding it into a quantum processor could optimise performance while mitigating some limitations associated with current quantum technologies.
As industries begin to recognise the transformative potential of quantum computing, investment in research and development is expected to surge. Collaborations between academia and industry will likely accelerate progress towards practical applications across sectors such as finance, healthcare, and logistics.
Ethical and Societal Implications of Quantum Computing with Qubits
The advent of quantum computing raises important ethical and societal considerations that must be addressed as technology progresses. One significant concern revolves around security; as quantum computers become capable of breaking widely used encryption methods, there is an urgent need for developing post-quantum cryptography standards that can withstand potential threats posed by powerful quantum algorithms like Shor’s algorithm. Additionally, the implications for privacy are profound.
Quantum computing could enable unprecedented data analysis capabilities that may infringe upon individual privacy rights if not managed responsibly. Policymakers must consider regulations that protect citizens from potential misuse while fostering innovation in this emerging field. Furthermore, there are concerns regarding access to quantum technology.
As with many technological advancements, there exists a risk that only certain entities—such as large corporations or governments—will have access to powerful quantum computing resources, potentially exacerbating existing inequalities in technology access and economic opportunity. In conclusion, while the journey towards fully realising the potential of qubits in quantum computing is fraught with challenges, it also presents an exciting frontier for scientific exploration and technological advancement. Addressing ethical considerations will be crucial in ensuring that the benefits of this revolutionary technology are shared equitably across society.
For businesses looking to navigate the complexities of data protection post-Brexit, appointing the right GDPR representative is crucial. This article provides valuable insights into the steps companies can take to ensure compliance with data regulations. Understanding customer behaviour and market trends is also essential for business success. If you’re interested in learning more about triangle patterns in trading, this article offers a comprehensive guide. Additionally, tracking customer satisfaction metrics can help boost loyalty and improve overall business performance. Check out this article for more information on how to enhance customer relationships.
FAQs
What is a qubit in quantum computing?
A qubit, short for a quantum bit, is the basic unit of information in quantum computing. It is the quantum analogue of a classical bit and can exist in multiple states simultaneously due to the principles of quantum superposition and entanglement.
How are qubits different from classical bits?
Classical bits can only exist in one of two states, 0 or 1, at any given time. Qubits, on the other hand, can exist in a superposition of both states simultaneously, allowing for much greater computational power and efficiency in quantum computing.
What is the role of qubits in quantum computing?
Qubits are the fundamental building blocks of quantum computers and are used to perform complex calculations and solve problems that are currently infeasible for classical computers. Their ability to exist in multiple states simultaneously allows quantum computers to process and store a vast amount of information in parallel.
How are qubits implemented in quantum computing systems?
Qubits can be implemented using various physical systems, such as superconducting circuits, trapped ions, and quantum dots. These systems are manipulated and controlled to create and maintain the quantum states of the qubits, enabling quantum computation.
What are the challenges in working with qubits?
One of the main challenges in working with qubits is maintaining their delicate quantum states, as they are highly susceptible to environmental noise and decoherence. Researchers are actively working on developing error correction techniques and improving qubit stability to overcome these challenges.